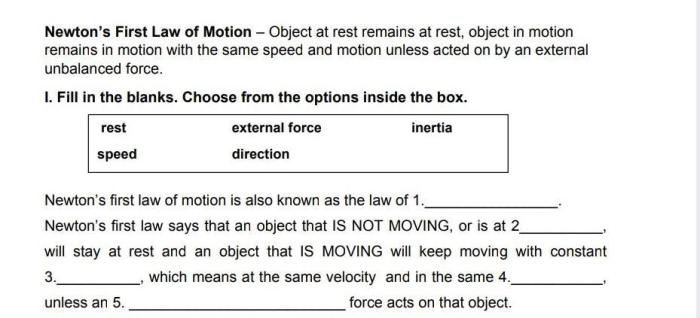
What is 2nd law of thermodynamics – What is the 2nd law of thermodynamics? It’s a fundamental principle in physics that governs the flow of energy and the direction of spontaneous processes. Imagine a cup of hot coffee left on a table. The coffee gradually cools down, transferring its heat to the surrounding air. This everyday phenomenon exemplifies the 2nd law, which states that the entropy of a closed system always increases over time.
Entropy is a measure of disorder or randomness within a system. The 2nd law implies that isolated systems naturally progress towards a state of greater disorder. This concept has profound implications for understanding the universe, from the evolution of stars to the functioning of biological organisms.
Key Concepts and Principles: What Is 2nd Law Of Thermodynamics
The Second Law of Thermodynamics is a fundamental principle in physics that governs the direction of energy transfer and the tendency of systems to become more disordered. It is closely related to the concept of entropy, a measure of the disorder or randomness within a system.
Heat Flow and Entropy
The Second Law of Thermodynamics states that heat always flows from a hotter object to a colder object, never the other way around. This spontaneous transfer of heat is driven by the increase in entropy of the system. Entropy is a measure of the number of possible arrangements of the particles in a system, and it always increases in an isolated system.
The more disordered a system is, the higher its entropy.
For example, if you place a hot cup of coffee on a table, heat will flow from the coffee to the cooler air and the table. As the coffee cools, its entropy decreases, but the entropy of the surrounding air and table increases by a greater amount. This ensures that the overall entropy of the system (coffee, air, and table) increases, which is in accordance with the Second Law of Thermodynamics.
Formulations of the Second Law
The Second Law of Thermodynamics can be expressed in different ways, each emphasizing a specific aspect of the principle. Two common formulations are the Clausius statement and the Kelvin-Planck statement.
Clausius Statement
The Clausius statement focuses on the impossibility of transferring heat from a colder body to a hotter body without any other effect. In simpler terms, it means that heat cannot spontaneously flow from a cold object to a hot object.
Kelvin-Planck Statement
The Kelvin-Planck statement focuses on the impossibility of creating a perpetual motion machine of the second kind. This type of machine would be able to continuously extract heat from a single reservoir and convert it entirely into work, without any other effect.
Irreversible Processes and Entropy
Irreversible processes are processes that cannot be reversed without leaving a change in the surroundings. These processes are characterized by an increase in entropy. For example, the mixing of two different gases is an irreversible process. Once the gases are mixed, they cannot be separated without expending energy.
Irreversible processes always result in an increase in the entropy of the universe.
The Second Law of Thermodynamics states that the entropy of an isolated system always increases or remains constant in an irreversible process. This means that the universe is constantly becoming more disordered, and it is impossible to create a system that becomes more ordered without increasing the disorder of its surroundings.
Applications of the Second Law
The Second Law of Thermodynamics is not merely a theoretical concept; it has profound implications for understanding and manipulating the world around us. Its principles govern various processes in engineering, chemistry, and biology, providing a framework for analyzing and predicting the behavior of systems.
Power Generation and Refrigeration
The Second Law plays a crucial role in the design and operation of power generation systems and refrigeration units. These systems rely on the transfer of heat between different temperatures, and the Second Law sets limits on the efficiency of these processes.
For example, in power plants, heat is generated from burning fuel and then used to produce steam, which drives turbines to generate electricity. The Second Law dictates that some heat energy will always be lost to the environment, limiting the efficiency of the power plant.
Similarly, refrigerators work by transferring heat from a cold reservoir (the inside of the refrigerator) to a hot reservoir (the surrounding environment). The Second Law sets a limit on the amount of heat that can be transferred per unit of work done, impacting the efficiency of the refrigerator.
Chemical Reactions and Spontaneity
The Second Law provides a framework for understanding the spontaneity of chemical reactions. A reaction is considered spontaneous if it occurs without external intervention, such as the addition of heat or pressure. The Second Law states that a spontaneous process always results in an increase in the entropy of the universe.
For instance, the combustion of fuels, such as wood or natural gas, is a spontaneous process that releases heat and light. The products of combustion, carbon dioxide and water, have a higher entropy than the reactants, fuel and oxygen. This increase in entropy drives the reaction forward.
The Gibbs free energy (G) is a thermodynamic potential that combines enthalpy (H) and entropy (S) to predict the spontaneity of a reaction at constant temperature and pressure. The change in Gibbs free energy (ΔG) for a reaction is given by:
ΔG = ΔH – TΔS
where T is the temperature in Kelvin.
If ΔG is negative, the reaction is spontaneous; if ΔG is positive, the reaction is non-spontaneous; and if ΔG is zero, the reaction is at equilibrium.
Biological Systems
The Second Law is fundamental to understanding the functioning of biological systems. Living organisms maintain a high degree of order and complexity, seemingly contradicting the Second Law’s tendency towards disorder. However, biological systems achieve this order by continuously exchanging energy and matter with their environment.
For instance, plants utilize sunlight to convert carbon dioxide and water into sugars and oxygen through photosynthesis. This process requires energy input from the sun and results in a decrease in entropy within the plant. However, the overall entropy of the universe increases due to the release of heat and the production of oxygen, a gas with higher entropy than carbon dioxide.
Similarly, animals consume food to obtain energy and maintain their complex structures. This process involves the breakdown of food molecules, releasing energy and increasing entropy. However, the overall entropy of the universe increases due to the release of heat and the production of waste products.
The Second Law provides a framework for understanding the flow of energy and matter in biological systems and the constraints that govern their organization and function.
Entropy and its Significance
Entropy is a fundamental concept in thermodynamics that measures the degree of disorder or randomness in a system. It is a state function, meaning its value depends only on the current state of the system and not on how it got there.
Entropy and Disorder
Entropy is directly related to the number of possible arrangements or microstates a system can have. The more microstates a system can access, the higher its entropy. For instance, a perfectly ordered crystal has a low entropy because its atoms are arranged in a highly specific and rigid structure. In contrast, a gas has a high entropy because its molecules can move freely and occupy a wide range of positions and orientations.
Entropy is a measure of the number of possible arrangements of the components of a system.
Changes in Entropy During Processes
Entropy changes during various processes, and the direction of change depends on whether the process increases or decreases the disorder of the system.
- Melting: When a solid melts into a liquid, the molecules gain more freedom of movement, leading to an increase in entropy. The liquid state has a higher entropy than the solid state.
- Boiling: Boiling involves a transition from a liquid to a gas, where molecules gain even more freedom of movement and occupy a much larger volume. This transition results in a significant increase in entropy.
- Mixing: Mixing two substances together increases the number of possible arrangements for the molecules, resulting in an increase in entropy. For example, mixing salt and water increases the entropy of the system.
Significance of Entropy in Spontaneous Processes
Entropy plays a crucial role in determining the direction of spontaneous processes, which are processes that occur naturally without any external intervention. The second law of thermodynamics states that the total entropy of an isolated system always increases over time for spontaneous processes.
The entropy of the universe is always increasing.
This principle implies that spontaneous processes tend to move towards a state of greater disorder. For example, a hot object will spontaneously transfer heat to a colder object until they reach thermal equilibrium. This process increases the entropy of the system because the heat energy is distributed more evenly, leading to a higher number of possible microstates.
Entropy is a fundamental concept that helps us understand the direction of natural processes and the tendency towards increasing disorder in the universe.
Examples of the Second Law in Action
The Second Law of Thermodynamics, while abstract, governs many familiar processes in our daily lives. From the simple act of cooking to the intricate workings of a refrigerator, the second law is at play, shaping the flow of energy and the direction of change. Understanding these examples helps us appreciate the profound implications of this fundamental law.
Everyday Examples
The Second Law of Thermodynamics manifests in everyday phenomena, often in subtle ways. Here are a few examples:
- Cooking: When you cook food, you are essentially increasing its entropy. Heat from the stove or oven transfers to the food, causing its molecules to move faster and become more disordered. The food changes state from raw to cooked, a process that increases entropy. This is why it’s difficult to reverse the cooking process; you can’t easily transform cooked food back into its raw state.
- Driving: A car engine converts chemical energy from fuel into mechanical energy to move the vehicle. However, this conversion is not perfectly efficient. A significant portion of the energy is lost as heat, contributing to the car’s overall entropy. This is why car engines get hot and why you need to cool them with a radiator.
- Refrigerator: A refrigerator, seemingly defying the second law, actually relies on it to function. It doesn’t create cold; it transfers heat from the inside to the outside. To do this, it uses a refrigerant that absorbs heat from the inside, becoming warmer. This warmer refrigerant then releases heat to the outside, effectively cooling the interior. The overall process increases the entropy of the system, but it achieves the desired effect of cooling the refrigerator’s contents.
Entropy and Energy Conversion Efficiency
Entropy plays a crucial role in the efficiency of energy conversion processes. Every energy conversion process involves some energy loss, usually as heat, which increases the entropy of the system. This means that no energy conversion process can be 100% efficient. The more energy is lost as heat, the lower the efficiency of the process.
For example, a power plant that burns coal to generate electricity loses a significant amount of energy as heat. This heat is released into the environment, increasing the entropy of the surroundings. The efficiency of a power plant is typically measured by its “Carnot efficiency,” which is limited by the temperature difference between the hot source (burning coal) and the cold sink (the environment). The greater the temperature difference, the higher the potential efficiency.
This concept of entropy and energy conversion efficiency is important for understanding the limitations of energy technologies and the need for sustainable energy solutions.
Historical Context
The second law of thermodynamics, a fundamental principle governing the behavior of energy and its transformations, has a rich and fascinating history. It evolved over centuries through the contributions of numerous scientists, each building upon the work of their predecessors. This journey reflects the evolution of scientific understanding and the power of observation and experimentation in shaping our knowledge of the natural world.
Key Contributors and Discoveries, What is 2nd law of thermodynamics
The development of the second law can be traced back to the 18th century, with key contributions from scientists who were investigating the efficiency of heat engines.
- Sadi Carnot (1796-1832), a French physicist, is considered the father of thermodynamics. His work, *Reflections on the Motive Power of Fire* (1824), laid the foundation for the second law by introducing the concept of a reversible heat engine and demonstrating that the efficiency of such an engine is limited by the temperature difference between the heat source and the heat sink. Carnot’s work established the theoretical framework for understanding the relationship between heat, work, and efficiency, which later formed the basis of the second law.
- James Prescott Joule (1818-1889), an English physicist, provided experimental evidence for the equivalence of heat and mechanical work. His experiments, conducted in the mid-19th century, demonstrated that mechanical work could be converted into heat and vice versa, establishing the principle of energy conservation and laying the groundwork for the first law of thermodynamics. Joule’s work further solidified the understanding of energy transformations, which was crucial for the development of the second law.
- Rudolf Clausius (1822-1888), a German physicist, made significant contributions to the formulation of the second law. He introduced the concept of entropy and formulated the first precise statement of the second law in 1850, stating that heat cannot spontaneously flow from a colder body to a hotter body. Clausius’s work was pivotal in defining the direction of energy flow and its implications for the universe.
- William Thomson, Lord Kelvin (1824-1907), a Scottish physicist, played a crucial role in the development of the second law. He extended Clausius’s work by formulating the second law in terms of the impossibility of creating a perpetual motion machine of the second kind. This formulation emphasized the irreversibility of natural processes and the limitations on energy conversion.
- James Clerk Maxwell (1831-1879), a Scottish physicist, applied statistical methods to thermodynamics, leading to the development of statistical mechanics. Maxwell’s work provided a microscopic interpretation of entropy and further solidified the understanding of the second law’s implications for the behavior of systems at the molecular level.
- Ludwig Boltzmann (1844-1906), an Austrian physicist, made significant contributions to statistical mechanics and the understanding of entropy. He proposed that entropy is a measure of the disorder or randomness of a system, and his work provided a deeper understanding of the second law’s implications for the evolution of the universe. Boltzmann’s work also helped to resolve the long-standing debate about the nature of entropy and its relationship to probability.
Timeline of Significant Discoveries
- 1824: Sadi Carnot publishes *Reflections on the Motive Power of Fire*, laying the foundation for the second law of thermodynamics.
- 1840s-1850s: James Prescott Joule conducts experiments demonstrating the equivalence of heat and mechanical work, establishing the principle of energy conservation.
- 1850: Rudolf Clausius formulates the first precise statement of the second law, stating that heat cannot spontaneously flow from a colder body to a hotter body.
- 1851: William Thomson, Lord Kelvin, proposes the impossibility of creating a perpetual motion machine of the second kind, providing another formulation of the second law.
- 1860s-1870s: James Clerk Maxwell develops statistical mechanics, providing a microscopic interpretation of entropy.
- 1870s-1890s: Ludwig Boltzmann makes significant contributions to statistical mechanics and the understanding of entropy, proposing that entropy is a measure of the disorder or randomness of a system.
Evolution of the Second Law
The second law of thermodynamics has evolved over time, becoming increasingly refined and encompassing. Early formulations focused on the limitations of heat engines and the direction of heat flow. Later developments, particularly those in statistical mechanics, provided a deeper understanding of entropy and its connection to probability and disorder. This evolution has led to a broader understanding of the second law’s implications for various fields, including chemistry, biology, and cosmology.
Ultimate Conclusion
The 2nd law of thermodynamics is a powerful concept that underpins our understanding of energy, matter, and the direction of change in the universe. It has far-reaching applications in various fields, from engineering and chemistry to biology and cosmology. As we continue to explore the intricacies of this law, we gain deeper insights into the fundamental workings of the world around us.
Popular Questions
What are some real-world examples of the 2nd law of thermodynamics?
Besides the coffee example, other examples include a hot iron cooling down, a glass of ice melting, and a balloon deflating. These processes all involve an increase in entropy.
Does the 2nd law of thermodynamics mean that everything is becoming more chaotic?
Not necessarily. The 2nd law applies to closed systems, but the universe is not a closed system. Local increases in order can occur within a system, as long as there is an overall increase in entropy elsewhere.
Can we violate the 2nd law of thermodynamics?
The 2nd law is a fundamental law of physics, and there is no known way to violate it. However, we can create systems that appear to violate the law by transferring entropy to another system, making the overall entropy increase.