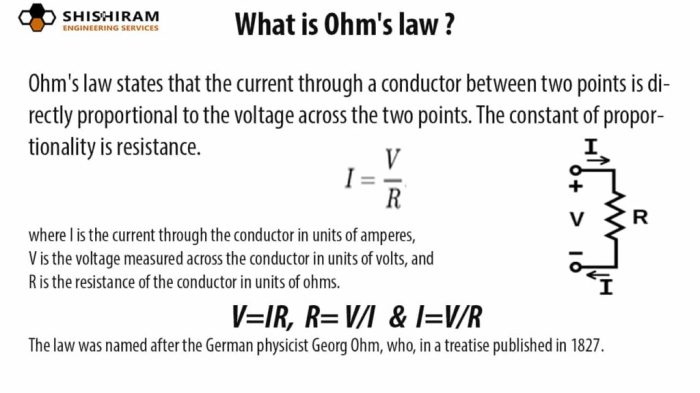
What is i in ohm’s law – What is ‘I’ in Ohm’s Law? This seemingly simple question opens the door to understanding one of the fundamental laws governing electricity. Ohm’s Law, named after the German physicist Georg Ohm, establishes a relationship between voltage, current, and resistance in a circuit. It’s a cornerstone of electrical engineering and plays a crucial role in everything from household appliances to complex electronic devices.
The symbol ‘I’ in Ohm’s Law represents electrical current, a measure of the flow of electric charge. Think of it as a river of electrons moving through a wire. The more electrons flowing, the greater the current. Current is measured in amperes (A), named after the French physicist André-Marie Ampère. The value of ‘I’ is influenced by factors like the voltage applied to the circuit and the resistance of the components within it.
The Role of ‘I’ in Ohm’s Law
Ohm’s Law is a fundamental principle in electrical circuits that describes the relationship between voltage, current, and resistance. One of the key components of this law is the symbol ‘I’, which represents electrical current.
Understanding Electrical Current
Electrical current is the flow of electric charge through a conductor. It is measured in amperes (A), named after the French physicist André-Marie Ampère. One ampere represents the flow of one coulomb of charge per second. The symbol ‘I’ in Ohm’s Law represents the magnitude of this electrical current.
Factors Influencing Current, What is i in ohm’s law
Several factors influence the value of current in a circuit. These factors are directly related to the components of Ohm’s Law:
Voltage (V): The electrical potential difference between two points in a circuit drives the flow of current. A higher voltage creates a stronger electrical force, resulting in a larger current.
Resistance (R): Resistance is the opposition to the flow of current. A higher resistance impedes the flow of charge, leading to a smaller current.
Practical Applications of Ohm’s Law
Ohm’s Law, a fundamental principle in electricity, is not just a theoretical concept but a powerful tool used in countless everyday applications. It helps us understand the relationship between voltage, current, and resistance in electrical circuits, allowing us to design, analyze, and troubleshoot various electrical systems.
Everyday Applications
Ohm’s Law is at work in numerous electrical systems we encounter daily. Here are some examples:
- Household Circuits: Our homes are wired with electrical circuits that deliver power to appliances and lighting. Ohm’s Law helps determine the appropriate wire gauge and fuse rating to ensure safe and efficient operation.
- Car Batteries: The battery in a car provides the electrical energy to start the engine. Ohm’s Law is crucial for understanding the battery’s voltage, current output, and internal resistance, which affect its performance and lifespan.
- Electronic Devices: From smartphones to computers, electronic devices rely on circuits governed by Ohm’s Law. It helps engineers design circuits that deliver the correct voltage and current to power various components.
Relationship between Voltage, Current, and Resistance
The following table illustrates the relationship between voltage, current, and resistance in different scenarios:
Scenario | Voltage (V) | Current (I) | Resistance (R) |
---|---|---|---|
Household Circuit (120V) | 120 | 10 | 12 |
Car Battery (12V) | 12 | 50 | 0.24 |
Smartphone Charger (5V) | 5 | 2 | 2.5 |
Troubleshooting Electrical Problems
Understanding Ohm’s Law is essential for troubleshooting electrical problems. By measuring voltage, current, and resistance, we can identify faulty components or circuits.
For instance, if a device is not receiving power, we can use Ohm’s Law to determine if the problem lies in the voltage source, the current path, or the resistance of the device itself.
Analogies for Understanding ‘I’ in Ohm’s Law: What Is I In Ohm’s Law
Analogies can be powerful tools for understanding abstract concepts like electrical current. By relating ‘I’ to familiar physical systems, we can gain a deeper intuition for how it behaves in circuits.
Water Flow Analogy
Imagine a garden hose connected to a faucet. The water pressure from the faucet represents voltage, the amount of water flowing through the hose represents current, and the size of the hose represents resistance.
- Higher water pressure (voltage) will cause more water (current) to flow through the hose.
- A larger hose (lower resistance) will allow more water (current) to flow at a given pressure (voltage).
- A smaller hose (higher resistance) will restrict the flow of water (current), even with high pressure (voltage).
This analogy helps visualize how voltage, current, and resistance are interconnected.
Traffic Flow Analogy
Consider a highway with a large number of cars. The speed limit represents voltage, the number of cars passing a point per minute represents current, and the number of lanes on the highway represents resistance.
- A higher speed limit (voltage) allows more cars (current) to pass a point per minute.
- A highway with more lanes (lower resistance) allows more cars (current) to pass a point per minute at a given speed limit (voltage).
- A highway with fewer lanes (higher resistance) restricts the flow of cars (current), even at a high speed limit (voltage).
This analogy highlights the relationship between voltage, current, and resistance in a real-world scenario.
Mechanical System Analogy
Think of a mechanical system with a rotating shaft connected to a load. The speed of the shaft represents voltage, the torque applied to the shaft represents current, and the friction between the shaft and its bearings represents resistance.
- A higher shaft speed (voltage) will result in a higher torque (current) applied to the load.
- Lower friction (lower resistance) will allow a higher torque (current) to be applied at a given shaft speed (voltage).
- Higher friction (higher resistance) will limit the torque (current) that can be applied, even at a high shaft speed (voltage).
This analogy connects electrical concepts to the familiar realm of mechanical systems.
The Significance of ‘I’ in Circuit Analysis
In the realm of electrical circuits, understanding the role of current (‘I’) is paramount. Current is the lifeblood of any circuit, driving the flow of charge and enabling the operation of various electrical devices. Determining the value of ‘I’ in a circuit is crucial for comprehending its behavior, optimizing its performance, and ensuring safe operation.
The Importance of Determining ‘I’ in Circuit Analysis
Current is a fundamental parameter that governs the behavior of electrical circuits. It dictates the amount of charge flowing through a conductor per unit time. Understanding the magnitude and direction of current is essential for:
- Circuit Design and Analysis: Determining the current flow in a circuit is a crucial step in circuit design and analysis. This information allows engineers to select appropriate components, predict circuit performance, and ensure safe operation.
- Component Selection: The current rating of components, such as resistors, capacitors, and transistors, determines the maximum current they can safely handle. Knowing the current flow in a circuit helps in selecting components that can withstand the expected current without failure.
- Troubleshooting and Repair: An abnormal current flow can indicate a fault in a circuit. Measuring the current can help identify the location of the fault and facilitate troubleshooting and repair.
- Power Calculations: Current is directly related to power dissipation in a circuit. Understanding the current flow allows engineers to calculate the power consumed by different components and optimize circuit efficiency.
The Influence of ‘I’ on Power Dissipation
Power dissipation in a circuit is the rate at which electrical energy is converted into other forms of energy, such as heat or light. The power dissipated by a component is directly proportional to the square of the current flowing through it. This relationship is described by the following equation:
P = I²R
where:
* P is the power dissipated (in watts)
* I is the current (in amperes)
* R is the resistance (in ohms)
This equation highlights the significant impact of current on power dissipation. As the current increases, the power dissipated by a component increases quadratically. This can lead to overheating and potential damage to the component if the current exceeds its rated value.
The Role of ‘I’ in Energy Consumption
Energy consumption in a circuit is the total amount of electrical energy used over a period of time. The energy consumed by a circuit is directly proportional to the current flowing through it and the time for which the current flows. This relationship is described by the following equation:
E = I²Rt
where:
* E is the energy consumed (in joules)
* I is the current (in amperes)
* R is the resistance (in ohms)
* t is the time (in seconds)
This equation demonstrates that current plays a crucial role in determining the energy consumption of a circuit. Higher current leads to greater energy consumption, which translates into higher electricity bills.
Applications of Ohm’s Law in Different Fields
Ohm’s Law, a fundamental principle in electrical engineering, governs the relationship between voltage, current, and resistance in an electrical circuit. Its widespread applicability extends beyond theoretical concepts, playing a crucial role in various fields, from electronics to medicine and research.
Electronics
Ohm’s Law is indispensable in electronics, underpinning the design and analysis of circuits. It helps determine the current flowing through a component, the voltage drop across it, or the resistance needed to achieve a desired outcome. For instance, in designing a circuit for a smartphone, engineers use Ohm’s Law to calculate the appropriate resistance for the power supply, ensuring the battery delivers the required current without overheating.
Telecommunications
Telecommunications heavily rely on Ohm’s Law for signal transmission and reception. The principle is applied in designing antennas, amplifiers, and other components used in transmitting and receiving electromagnetic waves. For example, in cellular networks, Ohm’s Law is used to calculate the impedance of the antenna, ensuring efficient signal transmission and reception.
Power Generation
Power generation systems rely on Ohm’s Law to control the flow of electricity. Generators produce electricity by converting mechanical energy into electrical energy. Ohm’s Law is used to calculate the resistance of the generator windings and the current flowing through them, ensuring efficient energy transfer.
Medical Devices and Instrumentation
Ohm’s Law finds applications in various medical devices and instrumentation. For instance, in electrocardiograms (ECGs), the principle is used to measure the electrical activity of the heart, providing valuable diagnostic information. Similarly, in blood glucose monitors, Ohm’s Law is applied to measure the resistance of a glucose solution, enabling the determination of blood sugar levels.
Research and Development
Ohm’s Law plays a vital role in research and development across various fields. Scientists use it to analyze electrical properties of materials, develop new electronic devices, and explore innovative technologies. For example, in nanotechnology, Ohm’s Law is used to study the electrical conductivity of nanomaterials, leading to the development of advanced electronic devices.
Limitations of Ohm’s Law
Ohm’s Law, a fundamental principle in electrical circuits, describes the relationship between voltage, current, and resistance. While it provides a powerful tool for understanding and analyzing circuits, it’s important to recognize that its applicability has certain limitations. These limitations arise from the fact that Ohm’s Law is based on an idealized model of a conductor, and real-world materials and circuits often deviate from this ideal behavior.
Non-Ohmic Materials
Ohm’s Law is based on the assumption that the resistance of a material remains constant regardless of the applied voltage. However, this is not always true. Some materials, known as non-ohmic materials, exhibit a resistance that changes with the applied voltage.
- Semiconductors: These materials, like silicon and germanium, are widely used in electronic devices. Their resistance decreases with increasing voltage, a characteristic that enables their use in transistors and diodes.
- Electrolytes: These are solutions containing ions that conduct electricity. Their resistance depends on the concentration of ions and the applied voltage. For example, the resistance of an electrolytic solution used in a battery decreases as the battery discharges.
- Gases: At low voltages, gases act as insulators. However, as the voltage increases, the gas molecules become ionized, increasing conductivity. This phenomenon is used in gas discharge lamps, such as neon lights.
The behavior of non-ohmic materials cannot be accurately predicted using Ohm’s Law. Instead, their current-voltage relationship is often described by more complex equations.
Limitations in Complex Circuits
While Ohm’s Law is effective for analyzing simple circuits with linear components, its application in complex circuits can be challenging.
- Non-Linear Components: Complex circuits often contain non-linear components like diodes, transistors, and operational amplifiers. These components have a non-linear relationship between voltage and current, making direct application of Ohm’s Law difficult.
- Inductive and Capacitive Elements: Circuits containing inductors and capacitors exhibit dynamic behavior, where current and voltage change over time. Ohm’s Law, which describes a static relationship, is not sufficient to analyze these circuits accurately.
- Time-Varying Conditions: In circuits with time-varying voltage or current sources, the resistance of components may also vary over time. This dynamic behavior cannot be captured by the static relationship described by Ohm’s Law.
For analyzing complex circuits, more advanced techniques like circuit analysis methods and numerical simulations are often required.
Conclusive Thoughts
Understanding the role of ‘I’ in Ohm’s Law is essential for anyone working with electricity. It allows us to analyze circuits, predict how they will behave, and troubleshoot problems. Whether you’re a seasoned electrical engineer or just curious about the world around you, Ohm’s Law provides a powerful framework for understanding the flow of electricity.
Query Resolution
How does ‘I’ relate to power in a circuit?
The power dissipated in a circuit is directly proportional to the square of the current (‘I’). This means that a higher current results in a greater power dissipation, which can lead to heat generation.
What are some examples of non-ohmic materials?
Some materials, like diodes and transistors, exhibit non-linear relationships between voltage and current. They don’t follow Ohm’s Law directly and their resistance can vary depending on the applied voltage.
How does ‘I’ affect the energy consumed by a circuit?
The energy consumed by a circuit is calculated by multiplying the power by the time the circuit is active. Since power is proportional to the square of the current, a higher current leads to greater energy consumption.